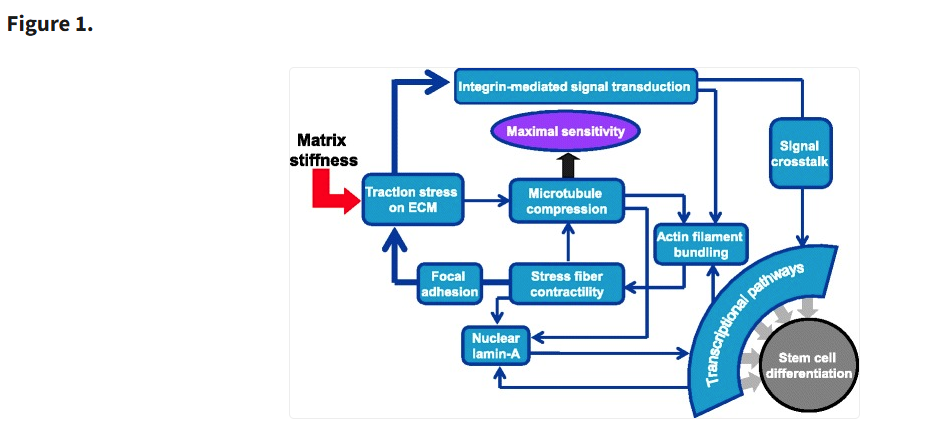
The Importance of Mechanical Load in Regulating Cellular Behavior
Mechanical loading is a fundamental factor in regulating cellular behavior, particularly in the context of stem cell proliferation, differentiation, and function. In native tissues, cells are continuously subjected to mechanical cues such as tension, compression, and shear forces, which influence their development and response to physiological stimuli. To replicate these biomechanical environments in vitro, dynamic bioreactor systems have been developed to apply controlled mechanical strain, enabling researchers to investigate mechanotransduction—the process by which cells convert mechanical stimuli into biochemical signals. Among these approaches, applying tensile strain to stem cells cultured on flexible membranes has proven to be a valuable method for simulating in vivo conditions, offering critical insights into how mechanical forces guide lineage specification and tissue regeneration. Understanding these mechanobiological interactions is essential for advancing tissue engineering, regenerative medicine, and the development of physiologically relevant in vitro models.
The Role of Mechanical Load in Stem Cell Differentiation
Applying mechanical load during stem cell culture can help maintain proliferative potential and extend the duration of the stem cell’s undifferentiated state by promoting cytoskeletal organization and reinforcing stemness-related signaling pathways. Controlled mechanical stimulation has been shown to regulate cellular aging processes by modulating key transcription factors and mechanotransductive pathways that influence self-renewal.
Additionally, mechanical load plays a crucial role in directing stem cell fate. For example, cyclic strain mimicking the mechanical environment of the developing heart can induce cardiac differentiation of pluripotent stem cells. Advanced bioreactors capable of precisely regulating cyclic loading parameters allow researchers to optimize mechanical cues for enhancing stem cell expansion while maintaining their regenerative potential. This capability opens new avenues for stem cell therapeutics by improving cell quality and functional differentiation, with broad applications in regenerative medicine and tissue engineering.
Application of Mechanical Load in Stem Cell Studies
The Flexcell® FX-6000™ Tension System is a widely used in vitro platform for studying the effects of mechanical loading on stem cell behavior by applying controlled tensile strain to cells cultured on flexible membranes. This system utilizes a vacuum-driven mechanism to create equibiaxial tensile strain, effectively mimicking physiological mechanical cues that cells experience in vivo. The use of vacuum technology, as opposed to electric motors, reduces the impact of electromagnetic fields and excess heat on cell cultures.
The mechanical loading mechanism involves placing a silicone elastomer membrane within a culture plate over a strain accessory centered on cylindrical loading posts. When negative pressure is applied, the vacuum pulls the membrane downward over the loading posts, inducing a uniform equibiaxial strain field at the center of each well. This controlled deformation mimics the stretching forces experienced by cells in tissues such as the dermis, myocardium, muscle, tendons, and cartilage. Conversely, when positive pressure is applied, the membrane relaxes back to its original state, enabling cyclic loading conditions that closely replicate dynamic tissue environments.
Using the FX-6000™ FlexSoft® program, researchers can design regimens that apply unconstrained, uniaxial, or equibiaxial tensile strain within specific strain parameters. Cyclic tensile strain can be applied with defined elongation percentages and frequencies to simulate physiological in vivo conditions. The strain field across the membrane can be precisely controlled via the FlexSoft® operating program, allowing researchers to explore various strain magnitudes and frequencies to determine optimal mechanical conditions for stem cell proliferation, differentiation, and function. Post-loading, researchers can assess cellular responses, including changes in gene expression, protein synthesis, and morphological adaptations.
To ensure proper setup, cells should be seeded onto a BioFlex® flexible-bottom 6-well culture plate, which is then secured within the rubber gaskets of the four-plate baseplate for a uniform vacuum seal. The Baseplate is placed in a standard incubator at 37°C and connected via Flex-in/Flex-out tubing to the FX-6000™ FlexLink® controller, where strain regimens are programmed with chosen waveform, frequency, and duration.
By utilizing the FX-6000™ Tension System, researchers can apply static or cyclic tensile strains to examine mechanotransduction pathways and investigate how mechanical forces influence cellular processes. For example, cyclic stretch has been shown to enhance cardiac differentiation of pluripotent stem cells by mimicking the rhythmic contractions of the developing heart. Similarly, mesenchymal stem cells (MSCs) can be directed toward osteogenic or tenogenic lineages depending on strain parameters. The ability to fine-tune mechanical cues in a controlled and reproducible manner makes the Flexcell FX-6000™ Tension System a valuable tool in stem cell research, regenerative medicine, and tissue engineering.
Importance of Substrate Stiffness in Stem Cell Culture
Substrate stiffness is a crucial factor in regulating stem cell behavior, influencing morphology, proliferation, and differentiation potential. Studies have demonstrated that softer substrates (0.1–1 kPa) promote neurogenic differentiation, resembling the elasticity of brain tissue, while intermediate stiffness substrates (8–17 kPa) favor myogenic differentiation, similar to muscle tissue mechanics. In contrast, stiffer substrates (25–40 kPa) support osteogenic differentiation, reflecting the rigidity of bone. These findings underscore the importance of tailoring substrate stiffness to achieve desired differentiation outcomes, making it a key consideration when passaging and expanding stem cells for therapeutic applications (see Lv H, et al., Stem Cell Res Ther. 2015).
Silicone elastomer membranes in cell culture plates facilitate the application of mechanical load to cells grown in vitro by providing a more flexible and physiologically relevant substrate. Unlike static hard polystyrene or glass cultureware, these membranes help prevent cellular stress and reduce substrate-induced cell shock, making them ideal for stem cell studies.
To further enhance these capabilities, Flexcell® has developed a new stem cell substrate product, CellSoft®, with tunable stiffness to match the in vivo stiffness of native tissues. CellSoft® substrates can be applied to round dishes, 6-well polystyrene plates, or flexible-bottom BioFlex® culture plates to enable mechanical loading of stem cells. Available in a variety of stiffness levels based on Young’s modulus, each batch of CellSoft® products is rigorously tested to ensure precise kPa stiffness, providing researchers with reliable and reproducible mechanical environments for in vitro stem cell culture.
Optimal Culture Plate Selection
The extracellular matrix (ECM) coating plays a vital role in promoting cell adhesion, growth, and morphology in vitro, as stem cells do not adhere or proliferate efficiently on rigid surfaces such as polystyrene. To create an optimal culture environment, it is essential to match substrate stiffness to the native mechanical properties of tissues and organs, ensuring conditions that closely mimic the in vivo environment.
Flexcell’s flexible-bottom culture plates come pre-coated with ECM proteins such as Amino, Collagen Type I, Collagen Type IV, and Laminin or are available untreated ready for custom ECM coatings. These coatings facilitate cell adhesion and growth, ensuring that stem cells maintain their structural integrity and differentiation potential. By integrating appropriate ECM coatings and substrate stiffness, researchers can optimize in vitro conditions for stem cell expansion, controlled differentiation, and functional maturity.
Benefits of Culturing Stem Cells on Soft Substrates
Culturing stem cells on soft substrates offers several advantages:
- Reduced Cellular Stress – Soft substrates better mimic the natural extracellular matrix of many tissues, minimizing cellular stress and reducing the risk of cell shock during in vitro culture.
- Enhanced Stemness Maintenance – Soft culture environments help maintain the stem-like phenotype of cells, preserving their proliferative capacity and multipotency, which is crucial for expanding stem cells before differentiation or therapeutic application (see Gerardo, et al., Sci Rep 9, 9086 (2019)).
- Improved Differentiation Efficiency – A substrate stiffness that closely resembles the native tissue environment provides a more conducive setting for differentiation, leading to more efficient and functional tissue-specific cell generation.
The application of mechanical loading using the Flexcell® dynamic bioreactor systems, combined with the selection of CellSoft® culture plates with tunable substrate stiffness, plays a pivotal role in directing stem cell behavior. These approaches are essential for advancing stem cell research by creating more physiologically relevant in vitro models, enabling a deeper understanding of disease mechanisms, enhancing tissue engineering strategies, and improving drug discovery and testing.